New technique allows researchers to perform more targeted imaging
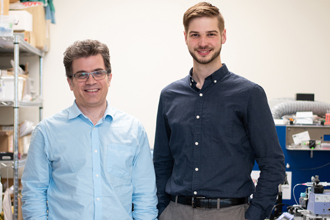
By Ana Gajic
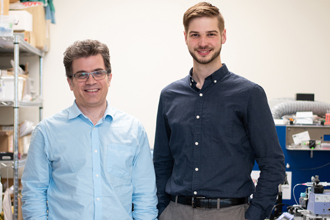
Dr. Michael Kolios (left) and Dr. Michael Moore
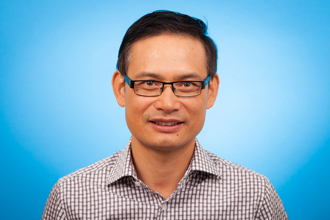
Dr. Xiao-Yan Wen
A new technique developed by scientists from iBEST and the Keenan Centre for Biomedical Science (KRCBS) allows researchers to perform more targeted photoacoustic imaging.
The development of size selective techniques could have an impact on disease diagnosis and treatment. In areas such as ophthalmology and neurosurgery, changes that occur at the sub-millimetre level can have consequences for patients. This research uncovers a new potential way to conduct highly specific imaging to observe these changes.
Published this week in Communications Physics, a group of researchers from iBEST— including Dr. Michael Moore, Eno Hysi, Muhannad Fadhel and Dr. Michael Kolios — teamed up with the KRCBS researchers in charge of the Zebrafish Centre for Advanced Drug Discovery: Dr. Xiao-Yan Wen, Dr. Susan El-Rass and Dr. Yonliang Xiao. Together, they set out to selectively visualize features of different sized biological cells and zebrafish.
We sat down with Dr. Moore to learn more about the team’s work in this area.
For your work in Communications Physics, what did you set out to study?
Photoacoustic imaging is quickly gaining attention in the field of biomedical research as affordable imaging that does not require the use of contrast agents. It is similar to ultrasound imaging in that both techniques use sound waves to construct images from inside a sample.
However, there is one key difference between the two approaches.
In ultrasound imaging, a sound wave is sent into a sample, scattered by internal structures, and the echoes from those structures are recorded by the detector and used to make an image. To put it succinctly: sound in, sound out. On the other hand, in photoacoustic imaging, light is instead sent into the sample. The light is absorbed by various structures, which causes them to heat up a fraction of a degree and undergo an almost imperceptible volume expansion. The sounds which are generated in the process (think of the rumbling of thunder after a flash of lightning) can then be recorded and used to create images of the objects from which they originated. Said another way, in photoacoustic imaging it’s light in, sound out.
With photoacoustic imaging it’s possible to visualize the location of structures that strongly absorb light, for example, blood vessels, within a sample of biological tissue. In the most common implementations, only the loudness of the photoacoustic waves is used to generate images, with louder sounds resulting in brighter image pixels. In this study, we wanted to see if we could develop a technique which instead used the pitch, or more specifically the frequency content, of the photoacoustic sound waves as a source of image contrast.
Why were you interested in this topic?
Similar to the way that different people have unique voices, the photoacoustic “sounds” produced by different objects — meaning the frequency content of those sounds — are also unique. In general, smaller objects tend to contain mostly high-frequency components, which are high-pitched sounds, while larger objects dominate at lower frequencies, which are low pitches.
We hypothesized that we could split the photoacoustic waves into different frequency bands (example, low, medium, high) and use each band separately to construct a unique image that would showcase objects of different sizes (example, large, medium, and small) accordingly. As many commonly imaged structures in the body have a wide distribution of sizes — such as veins, arteries and capillaries — we believed that such a technique would allow us to selectively accentuate features of specific size in photoacoustic images where they would otherwise be hidden or not as prominent.
What were your key findings?
By subdividing the frequency content of our recorded photoacoustic signals into different frequency bands, we were able to demonstrate selective enhancement of features of different sizes in samples ranging from single biological cells to live zebrafish larvae.
In biological cells, by tuning the frequency used to generate the final image we could selectively enhance either the appearance of the nucleoli within the cell nucleus, or the cytoplasm filling the rest of the cell without the use of dyes that would be mandatory in conventional imaging.
In the larval zebrafish, we demonstrated that we could individually showcase vessels of different sizes by again tuning into, and creating images from, different frequency bands present in the photoacoustic signals.
Why is this work important?
Every day our brains are bombarded with information from our senses. Through evolution, our minds have adapted so that they can filter out irrelevant or inconsequential input and keep only what is of use to us; the same way your email filters spam messages into your “junk” folder.
Our technique, which we have called F-Mode (for frequency mode), works in much the same way. By only selecting frequencies which contain information that is of interest to us, we can generate images that hone in on and depict exactly what we want to see.
We believe that our technique has potential for clinical translation since the segmentation and enhancement of features of different scale for disease diagnosis and treatment planning is of great importance in medical imaging. In particular, the development of techniques for selective enhancement of blood vessels of various scales has clinical applications in ophthalmology and neurosurgery. The detection of sub-millimetre changes in vessel diameter is an important indicator of conditions including hypertension.
What’s next for your research in this area?
Our ultimate goal is to apply the technique to photoacoustic images that have been acquired from humans to better visualize vessels of different sizes.
Going forward we will be investigating the use of other techniques — for example, machine learning — to further optimize the algorithm so minimal user input is required.
About St. Michael’s Hospital
St. Michael’s Hospital provides compassionate care to all who enter its doors. The hospital also provides outstanding medical education to future health care professionals in more than 29 academic disciplines. Critical care and trauma, heart disease, neurosurgery, diabetes, cancer care, care of the homeless and global health are among the Hospital’s recognized areas of expertise. Through the Keenan Research Centre and the Li Ka Shing International Healthcare Education Centre, which make up the Li Ka Shing Knowledge Institute, research and education at St. Michael’s Hospital are recognized and make an impact around the world. Founded in 1892, the hospital is fully affiliated with the University of Toronto.
About Unity Health Toronto
Unity Health Toronto, comprised of Providence Healthcare, St. Joseph’s Health Centre and St. Michael’s Hospital, works to advance the health of everyone in our urban communities and beyond. Our health network serves patients, residents and clients across the full spectrum of care, spanning primary care, secondary community care, tertiary and quaternary care services to post-acute through rehabilitation, palliative care and long-term care, while investing in world-class research and education. For more information, visit www.unityhealth.to.